Dysbiosis of gut microbiota in inflammatory bowel disease: Current therapies and potential for microbiota-modulating therapeutic approaches
DOI:
https://doi.org/10.17305/bjbms.2020.5016Keywords:
Inflammatory bowel disease, microbiota, dysbiosis, anti-TNF-α, microbiome-modulating therapyAbstract
There is a growing body of evidence reinforcing the unique connections between the host microbiome, health, and diseases. Due to the extreme importance of the symbiotic relationship between the intestinal microbiome and the host, it is not surprising that any alteration in the gut microbiota would result in various diseases, including inflammatory bowel disease (IBD), Crohn’s disease, (CD) and ulcerative colitis (UC). IBD is a chronic, relapsing-remitting condition that is associated with significant morbidity, mortality, compromised quality of life, and costly medical care. Dysbiosis is believed to exacerbate the progression of IBD. One of the currently used treatments for IBD are anti-tumor necrosis factor (TNF) drugs, representing a biologic therapy that is reported to have an impact on the gut microbiota composition. The efficacy of anti-TNF agents is hindered by the possibility of non-response, which occurs in 10-20% of treated patients, and secondary loss of response, which occurs in up to 30% of treated patients. This underscores the need for novel therapies and studies that evaluate the role of the gut microbiota in these conditions. The success of any therapeutic strategy for IBD depends on our understanding of the interactions that occur between the gut microbiota and the host. In this review, the health and disease IBD-associated microbiota patterns will be discussed, in addition to the effect of currently used therapies for IBD on the gut microbiota composition, as well as new therapeutic approaches that can be used to overcome the current treatment constraints.
Citations
Downloads
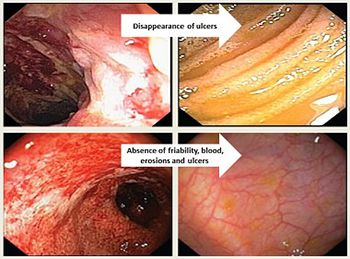
Downloads
Additional Files
Published
Issue
Section
Categories
How to Cite
Accepted 2020-09-17
Published 2021-06-01