The effects of platelet gel on cultured human retinal pigment epithelial (hRPE) cells
DOI:
https://doi.org/10.17305/bjbms.2017.2103Keywords:
Platelet gel, human retinal pigment epithelial cells, PAX6, cell viability, PG, hRPE cellsAbstract
The positive role of platelet gel (PG) in tissue regeneration is well known, however, other characteristics of PG still remain to be determined. We investigated cellular and molecular changes in cultured human retinal pigment epithelial (hRPE) cells when treated with different concentrations of PG named PG1, PG2, and PG3. hRPE cells were isolated from donor eyes of two newborn children, within 24 hours after their death. The cells were treated with three concentrations of PG for 7 days: 3 × 104/ml (PG1), 6 × 104/ml (PG2), and 9 × 104/ml (PG3). Fetal bovine serum was used as a control. Immunocytochemistry was performed with anti-RPE65 (H-85), anti-Cytokeratin 8/18 (NCL-5D3), and anti-PAX6 antibody. We used MTT assay to determine cell viability. Gene expressions of PAX6, MMP2, RPE65, ACTA2, MKI67, MMP9, and KDR were analyzed using real-time PCR. A significant increase in viability was observed for PG3-treated cells compared to control (p = 0.044) and compared to PG1 group (p = 0.027), on day 7. Cellular elongation together with dendritiform extensions were observed in PG-treated cells on days 1 and 3, while epithelioid morphology was observed on day 7. All cells were immunoreactive for RPE65, cytokeratin 8/18, and PAX6. No significant change was observed in the expression of MKI67 and PAX6, but the expressions of MMP2, MMP9, ACTA2, and KDR were significantly higher in PG2-treated cells compared to controls (p < 0.05). Our results indicate that increased concentration of PG and extended exposure time have positive effects on viability of hRPE cells. PG may be useful for hRPE cell encapsulation in retinal cell replacement therapy.
Citations
Downloads
References
The Eye M.D. Association. Retina and retinal pigment epithelium. In: Ophthalmic Pathology and Intraocular Tumors. Basic and Clinical Science Course. 2016-2017th ed. San Francisco: American Academy of Ophthalmology; 2016. p. 148.
Klein R, Chou CF, Klein BE, Zhang X, Meuer SM, Saaddine JB. Prevalence of age-related macular degeneration in the US population. Arch Ophthalmol 2011;129(1):75-80. https://doi.org/10.1001/archophthalmol.2010.318.
Mehta S. Age-related macular degeneration. Prim Care 2015;42(3):377-91. https://doi.org/10.1016/j.pop.2015.05.009.
Alexander P, Thomson HA, Luff AJ, Lotery AJ. Retinal pigment epithelium transplantation: Concepts, challenges, and future prospects. Eye (Lond) 2015;29(8):992-1002. https://doi.org/10.1038/eye.2015.89.
Forest DL, Johnson LV, Clegg DO. Cellular models and therapies for age-related macular degeneration. Dis Model Mech 2015;8(5):421-7. https://doi.org/10.1242/dmm.017236.
Pasovic L, Eidet JR, Lyberg T, Messelt EB, Aabel P, Utheim TP. Antioxidants improve the viability of stored adult retinal pigment epithelial-19 cultures. Ophthalmol Ther 2014;3(1-2):49-61. https://doi.org/10.1007/s40123-014-0024-9.
Ho W, Tawil B, Dunn JC, Wu BM. The behavior of human mesenchymal stem cells in 3D fibrin clots: Dependence on fibrinogen concentration and clot structure. Tissue Eng 2006;12(6):1587-95. https://doi.org/10.1089/ten.2006.12.1587.
Duong H, Wu B, Tawil B. Modulation of 3D fibrin matrix stiffness by intrinsic fibrinogen-thrombin compositions and by extrinsic cellular activity. Tissue Eng Part A 2009;15(7):1865-76. https://doi.org/10.1089/ten.tea.2008.0319.
van den Dolder J, Mooren R, Vloon AP, Stoelinga PJ, Jansen JA. Platelet-rich plasma: Quantification of growth factor levels and the effect on growth and differentiation of rat bone marrow cells. Tissue Eng 2006;12(11):3067-73. https://doi.org/10.1089/ten.2006.12.3067.
Jalowiec JM, D'Este M, Bara JJ, Denom J, Menzel U, Alini M, et al. An in vitro investigation of platelet-rich plasma-gel as a cell and growth factor delivery vehicle for tissue engineering. Tissue Eng Part C Methods 2016;22(1):49-58. https://doi.org/10.1089/ten.tec.2015.0223.
Najafabadi HS, Soheili ZS, Ganji SM. Behavior of a spontaneously arising human retinal pigment epithelial cell line cultivated on thin alginate film. J Ophthalmic Vis Res 2015;10(3):286-94. https://doi.org/10.4103/2008-322X.170357.
Moroz A, Bittencourt RA, Felisbino SL, da Rosa Pereira H, Rossi-Ferreira R, Deffune E. Platelet gel: 3D scaffold for cell culture. Acta Ortop Bras 2009;17(2):43-5. https://doi.org/10.1590/S1413-78522009000200008.
Heller JP, Martin KR. Enhancing RPE cell-based therapy outcomes for AMD: The role of Bruch's membrane. Transl Vis Sci Technol 2014;3(3):11. https://doi.org/10.1167/tvst.3.4.4.
Falkner-Radler CI, Krebs I, Glittenberg C, Povazay B, Povazay B, Drexler W, et al. Human retinal pigment epithelium (RPE) transplantation: Outcome after autologous RPE choroid sheet and RPE cell-suspension in a randomised clinical study. Br J Ophthalmol 2011;95(3):370-5. https://doi.org/10.1136/bjo.2009.176305.
Binder S. Scaffolds for retinal pigment epithelium (RPE) replacement therapy. Br J Ophthalmol 2011;95(4):441-2. https://doi.org/10.1136/bjo.2009.171926.
Afshari FT, Kwok JC, Andrews MR, Blits B, Martin KR, Faissner A, et al. Integrin activation or alpha 9 expression allows retinal pigmented epithelial cell adhesion on Bruch's membrane in wet age-related macular degeneration. Brain 2010;133(Pt 2):448-64. https://doi.org/10.1093/brain/awp319.
Xie X, Wang Y, Zhao C, Guo S, Liu S, Jia W, et al. Comparative evaluation of MSCs from bone marrow and adipose tissue seeded in PRP-derived scaffold for cartilage regeneration. Biomaterials 2012;33(29):7008-18. https://doi.org/10.1016/j.biomaterials.2012.06.058.
Campochiaro PA, Hackett SF, Vinores SA, Freund J, Csaky C, LaRochelle W, et al. Platelet-derived growth factor is an autocrine growth stimulator in retinal pigmented epithelial cells. J Cell Sci 1994;107(Pt 9):2459-69.
Booij JC, Baas DC, Beisekeeva J, Gorgels TG, Bergen AA. The dynamic nature of Bruch's membrane. Prog Retin Eye Res 2010;29(1):1-18. https://doi.org/10.1016/j.preteyeres.2009.08.003.
Das A, Frank RN, Zhang NL, Turczyn TJ. Ultrastructural localization of extracellular matrix components in human retinal vessels and Bruch's membrane. Arch Ophthalmol 1990;108(3):421-9. https://doi.org/10.1001/archopht.1990.01070050119045.
Ho TC, Del Priore LV. Reattachment of cultured human retinal pigment epithelium to extracellular matrix and human Bruch's membrane. Invest Ophthalmol Vis Sci 1997;38(6):1110-8.
Han J, Yan XL, Han QH, Li YJ, Du ZJ, Hui YN. Integrin ß1 subunit signaling is involved in the directed migration of human retinal pigment epithelial cells following electric field stimulation. Ophthalmic Res 2011;45(1):15-22. https://doi.org/10.1159/000313552.
Tezel TH, Del Priore LV. Reattachment to a substrate prevents apoptosis of human retinal pigment epithelium. Graefes Arch Clin Exp Ophthalmol 1997;235(1):41-7. https://doi.org/10.1007/BF01007836.
Moroz A, Deffune E. Platelet-rich plasma and chronic wounds: Remaining fibronectin may influence matrix remodeling and regeneration success. Cytotherapy 2013;15(11):1436-9. https://doi.org/10.1016/j.jcyt.2013.05.019.
Li H, Usas A, Poddar M, Chen CW, Thompson S, Ahani B, et al. Platelet-rich plasma promotes the proliferation of human muscle derived progenitor cells and maintains their stemness. PLoS One 2013;8(6):e64923. https://doi.org/10.1371/journal.pone.0064923.
Rubio-Azpeitia E, Andia I. Partnership between platelet-rich plasma and mesenchymal stem cells: In vitro experience. Muscles Ligaments Tendons J 2014;4(1):52-62. https://doi.org/10.11138/mltj/2014.4.1.052.
Giusti I, D'Ascenzo S, Mancò A, Di Stefano G, Di Francesco M, Rughetti A, et al. Platelet concentration in platelet-rich plasma affects tenocyte behavior in vitro. Biomed Res Int 2014;2014:630870. https://doi.org/10.1155/2014/630870.
Hotary KB, Yana I, Sabeh F, Li XY, Holmbeck K, Birkedal-Hansen H, et al. Matrix metalloproteinases (MMPs) regulate fibrin-invasive activity via MT1-MMP-dependent and -independent processes. J Exp Med 2002;195(3):295-308. https://doi.org/10.1084/jem.20010815.
Eichler W, Friedrichs U, Thies A, Tratz C, Wiedemann P. Modulation of matrix metalloproteinase and TIMP-1 expression by cytokines in human RPE cells. Invest Ophthalmol Vis Sci 2002;43(8):2767-73.
Moroz A, Delella FK, Lacorte LM, Deffune E, Felisbino SL. Fibronectin induces MMP2 expression in human prostate cancer cells. Biochem Biophys Res Commun 2013;430(4):1319-21. https://doi.org/10.1016/j.bbrc.2012.12.031.
Moroz A, Felisbino SL, Deffune E. Platelet and plasma bioactive scaffolds for stem cell differentiation: What are we missing? Platelets 2014;25(7):556-7. https://doi.org/10.3109/09537104.2013.836748.
Wolf G. Function of the protein RPE65 in the visual cycle. Nutr Rev 2005;63(3):97-100. https://doi.org/10.1111/j.1753-4887.2005.tb00127.x.
Kiser PD, Palczewski K. Membrane-binding and enzymatic properties of RPE65. Prog Retin Eye Res 2010;29(5):428-42. DOI: 10.1016/j.preteyeres.2010.03.002.
Kiser PD, Zhang J, Badiee M, Li Q, Shi W, Sui X, et al. Catalytic mechanism of a retinoid isomerase essential for vertebrate vision. Nat Chem Biol 2015;11(6):409-15. https://doi.org/10.1038/nchembio.1799.
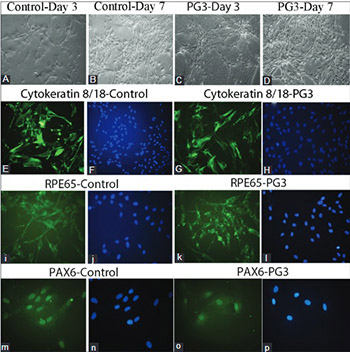
Downloads
Additional Files
Published
Issue
Section
Categories
How to Cite
Accepted 2017-05-15
Published 2017-11-20